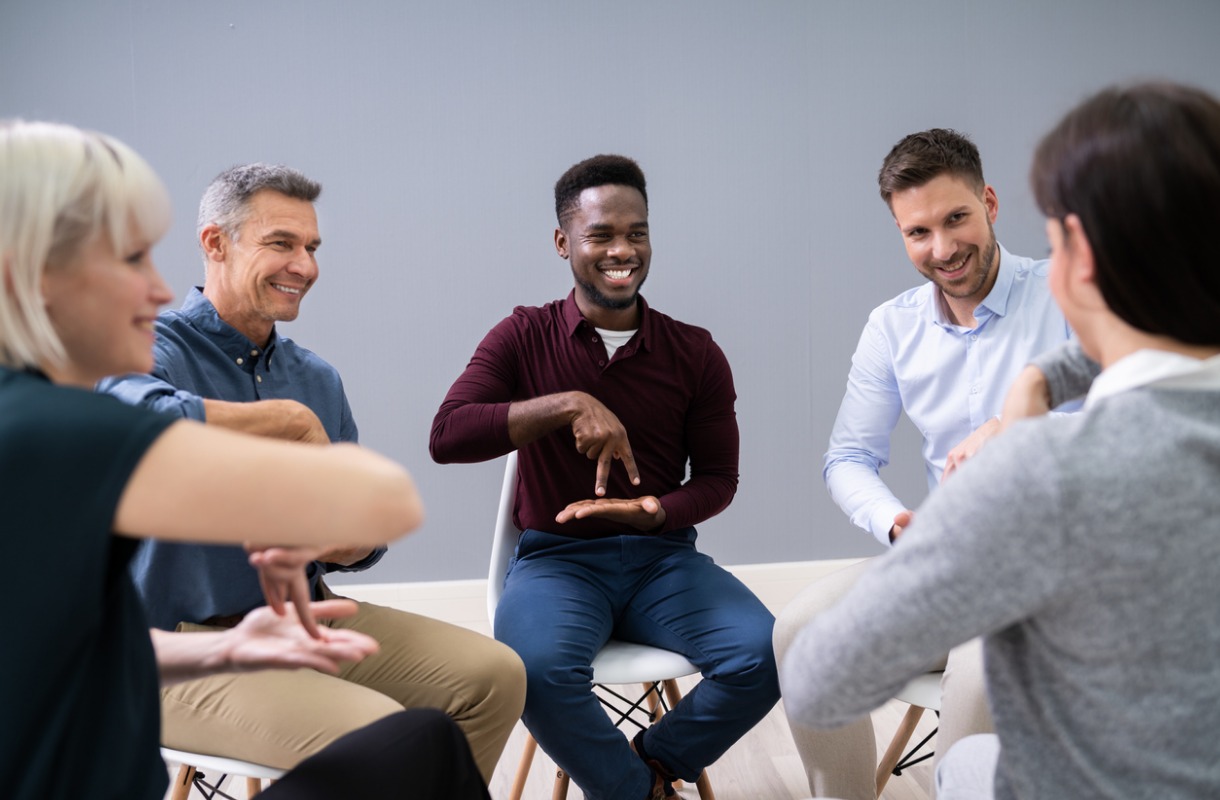
Issue 3 | Article 1
Abstract
Traditional teaching methodologies have focused on enhancing the knowledge and skills of people with good reading, movement, and verbal communication abilities. Due mainly to technological limitations and cost considerations, they have largely ignored people who do not possess these abilities. However, impressive results from three neuroscience experiments conducted in 2021 using Brain-Computer-Interface (BCI) technologies offer the prospect of vastly improved educational opportunities for those with impaired vision, movement, and speech.
Introduction
In recent decades, higher education providers have enhanced considerably the content and delivery of materials and learning activities for able-bodied students, particularly those with good vision, movement, and speaking ability. The enhancements have been led by improvements in technology, notably development of the hardware and software used in computers and mobile phones. However, taking advantage of these enhancements requires that students can read textbooks and other instructional materials; can participate in experiments requiring the movement and/or operation of physical objects such as chemicals, syringes, and machinery; and can communicate easily with their teachers and fellow students. Wheel chairs, talking books, and personal tutors have provided some help to disadvantaged students, but this help has been limited and available to only a very small proportion of the disabled population. Recent developments in BCI technologies indicate that much greater help may soon be available.
Humans controlling electronic devices solely with their thoughts may seem a futuristic concept, but BCI technologies are swiftly turning the concept into tangible realities (Kawala-Sterniuk et al., 2021). A BCI serves as a translator that decodes a brain signal about an intended action into a command that a computer can understand and execute, thus replacing a natural route that a brain signal normally takes through a human neuromuscular system to body parts (Shih et al., 2012). An invasive BCI is a method by which an electrode is surgically positioned on a cerebral cortex to send brain signals to the electrocorticogram (ECoG) to read. Invasive BCIs provide reliable and accurate data, which can be analysed to decipher vision, movement, and speech (Kawala-Sterniuk et al., 2021).
This article looks at three barriers to learning for physically disadvantaged people and three significant advances in BCI technologies in 2021 that promise to reduce the impact of these barriers.
Vision
It is estimated that, globally in 2020, six million people were totally blind (zero light perception), another 34 million were legally blind (central visual acuity of 20/200 or less in the better eye even when wearing glasses or contact lenses), and a further 240 million had moderately to severely impaired vision. The incidence of total and legal blindness increased dramatically with age, rising from 1% for those in their 50s to 4% for people in their 70s and almost 10% for those in their 80s and above. The proportion of the population with impaired vision was about five times higher in the low-income areas of the Middle East, West Asia, and Sub-Saharan Africa than in the high-income regions of Australasia, the United States, and Western Europe. The number of blind people is forecast to increase to 55 million in 2030 and 115 million by 2050, with proportionate increases projected for the population with moderately and severely impaired vision.
In 2021, a team of researchers from The University of Utah and other institutions led by Eduardo Fernandez combined the Utah Electrode Array (UEA) with a pair of camera glasses in an experiment that allowed 57-year-old Berna Gomez, who had not had “light perception” for 16 years, “to discriminate complex patterns”, “identify some letters and recognize object boundaries” (Fernández et al., 2021). Unlike retinal implants that use artificial light pulses for optic nerve stimulation (Mills, Jalil, Stanga, 2017), the UEA transmits visual information directly from the camera glasses to the brain, bypassing the optic nerve altogether. By using just one UEA containing 96 electrodes, the scientists were able to demonstrate both the safety and efficacy of the new technology. They are confident that use of 7-10 UEAs would allow restoration of “functional vision in the blind” (Fernández et al., 2021). This would permit students who are blind to read adaptive versions of written materials in courses as well as distinguish objects and processes demonstrated in disciplines such as medicine and engineering.
Writing
In America, approximately 2% of the population live with paralysis – defined as a disorder in a person’s central nervous system that results in difficulty or inability to move the upper or lower extremities. The main causes are stroke (34%), spinal cord injury (27%) and multiple sclerosis (19%). More than 40% of those living with paralysis are unable to work, and of those who can work only 25% are employed. Approximately a third of households with a paralysed person earn a household income that is less than 10% of the national average.
About one in 100 Americans has moderate to severe mobility impairment of their arms and hands. This impacts their ability to undertake personal activities such as dressing, bathing, and self-care. It also impacts their ability to write by hand or on a computer. They are therefore unable to take notes during lectures or to provide written answers to exam questions. Further, writing is not merely a vehicle for recording information, but also an activity that focuses the writer’s attention, facilitates deeper consideration of facts and ideas, and generates new ideas. Many students learn and create while they write.
In 2021, a research team from Stanford University conducted an experiment using two sensors, each carrying 100 electrodes (Sample, 2021), and a new approach to decoding imaginary handwriting movements. Their method enabled a participant with a paralyzed hand to type 90 characters per minute at 94.1% raw-accuracy rate (Willett et al., 2021). This was more-than double the previous best performance of 40 characters per minute using BCI-based point-and-click typing with a computer cursor. Since the typing speed of healthy smartphone users is about 115 characters per minute, the scientists predict a “bright” future for this new BCI technique (Willett et al., 2021). Interestingly for handwriting, the study also demonstrated that complex patterns of movement are easier to decrypt than straight-line movements.
Speech
Approximately 3% of Americans have difficulty using their voices. They may stutter; have little control over pitch, loudness, or quality; or be unable to form speech at all. The causes include genetic factors, workplace and other injuries, surgery (all of which can lead to vocal cord paralysis) and advancing age. The disability can discourage intelligent people from pursuing higher education, participating actively in tutorials, and engaging in individual discussions with their lecturers and other students.
In 2021, a group of scientists from the University of California San Francisco (UCSF) used an implant consisting of 128 electrodes together with newly created speech-detection and word-classification models to enable a paralysed man to project 18 words per minute onto a screen with 93% accuracy by attempting to say them (Moses et al., 2021). This clinical research trial represents the first successful demonstration of direct decoding of speech from brain activity (Marks, 2021). The development group feels that by being more natural and requiring less effort than cursor-based, letter-by-letter selection interfaces (Moses et al., 2021), and having a vocabulary range of 50 words already, this BCI system shows “strong promise” of returning a voice to those who have lost the ability to speak without assistance (Marks, 2021).
BCI: Microchips
To move the development of BCI forward, scientists need electrode sensors that can collect data from larger brain areas (Stacey, 2021). Researchers at Brown University are responding to this challenge by working on what they call “neurograins”. While most current BCIs are based on monolithic electrode arrays that have limitations in terms of electrode placement and scaling, neurograins create a wireless network of individual microchips that can be spread across a wide brain area (Lee, Leung, Lee et al., 2021). Having already successfully recorded brain signals from a rat’s cerebral cortex using 48 neurograins, the Brown University team expects to scale the technology up to 770 neurograins (Lee, Leung, Lee et al., 2021), providing scientists with unprecedented views of the brain (Stacey, 2021).
Conclusion
Although the latest discoveries in vision, movement, and speech BCIs were experiential studies, tested on only one participant in each, they have revealed the breathtaking potential of BCI technologies. With the current exponential growth of technological knowledge and with more participants being involved in BCI research across an increasing number of countries, it is easy to foresee a rapidly growing role for BCIs in providing new devices to facilitate vision, writing, and speaking by people with disabilities. These new devices will promote equity and inclusivity by making education available to a larger proportion of the global population.
References
Kawala-Sterniuk, A., Browarska, N., Al-Bakri, A., Pelc, M., Zygarlicki, J., Sidikova, M., Martinek, R., & Gorzelanczyk, E. J. (2021). Summary of over Fifty Years with Brain-Computer Interfaces-A Review. Brain sciences, 11(1), 43. https://doi.org/10.3390/brainsci11010043.
Shih, J. J., Krusienski, D. J., & Wolpaw, J. R. (2012). Brain-computer interfaces in medicine. Mayo Clinic proceedings, 87(3), 268–279. https://doi.org/10.1016/j.mayocp.2011.12.008.
Clerc M., Bougrain L., Lotte F. (2016). Brain-Computer Interfaces 2: Technology and Applications. ISTE Ltd and John Wiley & Sons, Inc.
Gonfalonieri A. (2020). What Brain-Computer Interfaces Could Mean for the Future of Work. Harvard Business Review accessed on 18 March 2022 from https://hbr.org/2020/10/what-brain-computer-interfaces-could-mean-for-the-future-of-work.
Sample I. (2005). Meet the mind readers. The Guardian, accessed on 18 March 2022 from https://www.theguardian.com/science/2005/mar/31/health.society.
Fernández E., Alfaro A., Soto-Sánchez C. et al. (2021). Visual precepts evoked with an intracortical 96-channel microelectrode array inserted in human occipital cortex. J Clin Invest; 131 (23). https://www.jci.org/articles/view/151331.
Mills, J., Jalil, A. & Stanga, P. 2017. Electronic retinal implants and artificial vision: journey and present. Eye, 31, 1383–1398 (2017). https://doi.org/10.1038/eye.2017.65.
Sample I. (2021). Paralysed man uses ‘mindwriting’ brain computer to compose sentences. The Guardian, accessed on 18 March 2022 from https://www.theguardian.com/science/2021/may/12/paralysed-man-mindwriting-brain-computer-compose-sentences.
Willett, F. R., Avansino, D. T., Hochberg, L. R., Henderson, J. M., & Shenoy, K. V. 2021. High-performance brain-to-text communication via handwriting. Nature, 593(7858), 249–254. https://doi.org/10.1038/s41586-021-03506-2.
Moses D. A., Metzger S. L., Liu J. R., et al. 2021. Neuroprosthesis for Decoding Speech in a Paralyzed Person with Anarthria. The New England Journal of Medicine, 385:217-227, https://www.nejm.org/doi/full/10.1056/NEJMoa2027540.
Marks R. 2021. “Neuroprosthesis” Restores Words to Man with Paralysis. UCSF Edu, accessed on 18 March 2022 from https://www.ucsf.edu/news/2021/07/420946/neuroprosthesis-restores-words-man-paralysis.
Stacey K. 2021. Researchers take step toward next-generation brain-computer interface system. Brown University accessed on 18 March 2022 from https://www.brown.edu/news/2021-08-12/neurograins.
Lee J., Leung V., Lee A. H., et al. 2021. Neural recording and stimulation using wireless networks of microimplants. Nat Electron 4, 604–614. https://doi.org/10.1038/s41928-021-00631-8.
BIOGRAPHIES
Valeriya Sytnik has an honours degree in International Business Management from the University of Nottingham, Ningbo, China. She is a Fellow of the Centre for Scholarship and Research (CSR) at UBSS. Valeriya has been working as a marketing manager for her family trading company, Tramplin (HK) Innovation Co. Limited, since 2011. She is currently on her way to pursue her second degree in psychology. Her research interests focus on social psychology of conflict analysis and resolution.
Angus Hooke is Emeritus Professor, Senior Scholarship Fellow, and Co-Director of the Centre for Scholarship and Research (CSR) at UBSS. His earlier positions include Division Chief in the IMF, Chief Economist at BAE (now ABARE), Chief Economist at the NSW Treasury, Professor of Economics at Johns Hopkins University, and Head of the Business School (3,300 students) at the University of Nottingham, Ningbo, China. Angus has published 11 books and numerous refereed articles in prestigious academic journals.